Your cart is currently empty!
Unveiling the Power of Lead-Carbon Technology Batteries: A Comprehensive Guide
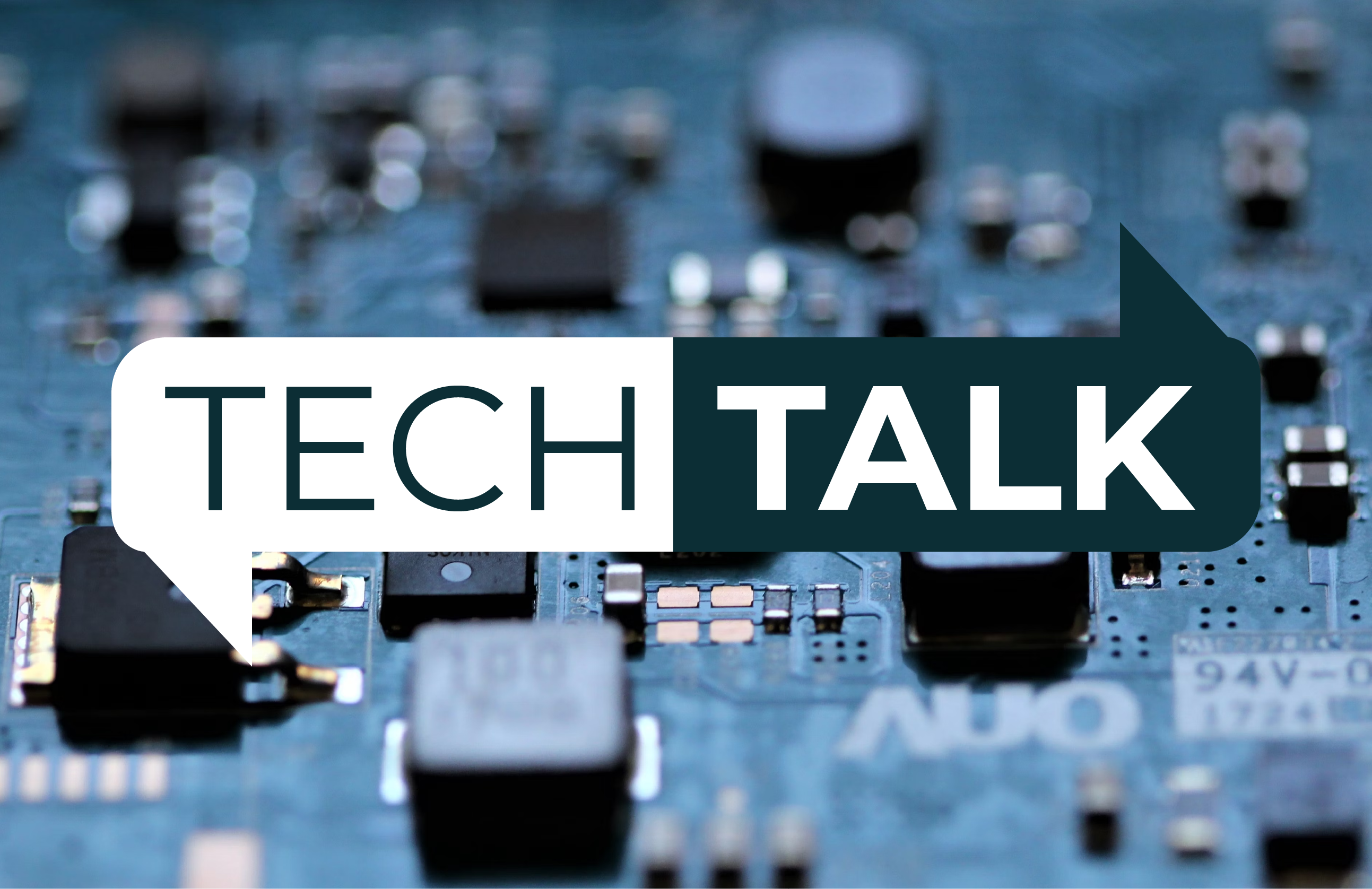
Everyone knows about lead-acid batteries and most are aware of lithium, but if lead-carbon still has you scratching your head, this talk is for you. With growing efforts to move away from fossil fuels, creativity in the renewable energy & battery storage sphere has resulted in the melding of age old technology in innovative ways. The addition of carbon to familiar lead-acid battery technology has delivered a low-cost and sustainable new product to the consumer battery market.
If you really enjoy a deep dive into fine grain tech, you’ll love the ‘Construction of Lead-Carbon Batteries’ section, but if you prefer to keep it a bit more general, cruise on down to the ‘Benefits of Lead-Carbon Batteries’ section.
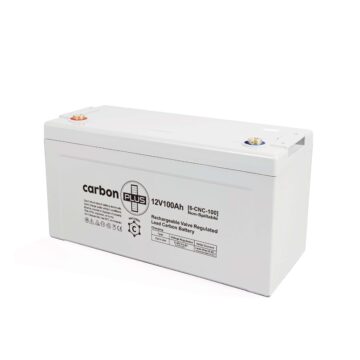
Understanding Lead-Carbon Batteries
Lead-carbon batteries have gained prominence due to their ability to provide sustainable and cost-effective energy storage solutions. To achieve their benefits, lead-carbon batteries employ a hybrid design that combines traditional lead-acid components with high-surface-area carbon materials.
Construction of Lead-Carbon Batteries
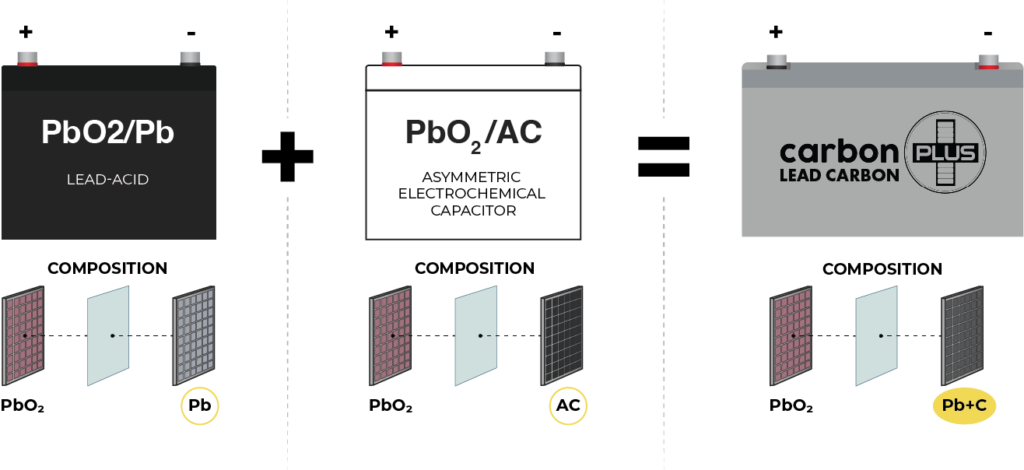
Fig.1. Lead-carbon battery configuration. Adapted from [1]
The construction of lead-carbon batteries involves modifying current LAB (Lead-Acid Battery) technology. The incorporation of activated carbon (AC) into the negative electrode enhances charge power and transforms the lead-acid battery into a lead-carbon battery. Lead-carbon electrodes, often referred to as LCBs, consist of a carbon-enhanced bifunctional lead-carbon composite negative electrode.
Key components inside a lead-carbon battery include sponge lead dendrites and AC particles connected in the negative active mass (NAM). This intricate structure enhances power and cycle life under partial state of charge (PSoC) operation. The addition of functional carbon materials addresses sulfation issues by increasing the conductivity of negative plates and providing additional active surfaces for lead sulfate (PbSO4) particles.
The inner structure of lead-carbon negative electrodes resembles a micro-ultrabattery negative plate, addressing challenges like parasitic hydrogen evolution reaction (HER), self-discharge, and electrode expansion during charge-discharge processes. Various carbon materials, such as carbon black, carbon nanotubes, graphene, and carbon nanofibers, are used as additives to enhance charge acceptance. The utilization of AC, known for its high specific capacitance, contributes to enhanced charge acceptance, even though it does not directly contribute to the capacitance of the lead-carbon electrode.
To counteract issues like parasitic HER induced by the exposure of carbon in the microstructure of lead-carbon to electrolytes, HER inhibitors (e.g., Zn, Ga, Bi, In, and Pb) are employed. A high affinity between lead and carbon components is essential for establishing a robust lead-carbon binary composite electrode. Despite the seemingly simple components, the lead-carbon composite electrode proves to be a sophisticated structure, requiring extensive research and design efforts for renewable energy storage and HEVs.
In the next section, we will delve into the mechanistic study and technological advancements of lead-carbon electrodes. Additionally, large-scale synthesis strategies of lead-carbon composite additives with potential applications in the NAM of commercial LCBs will be explored.
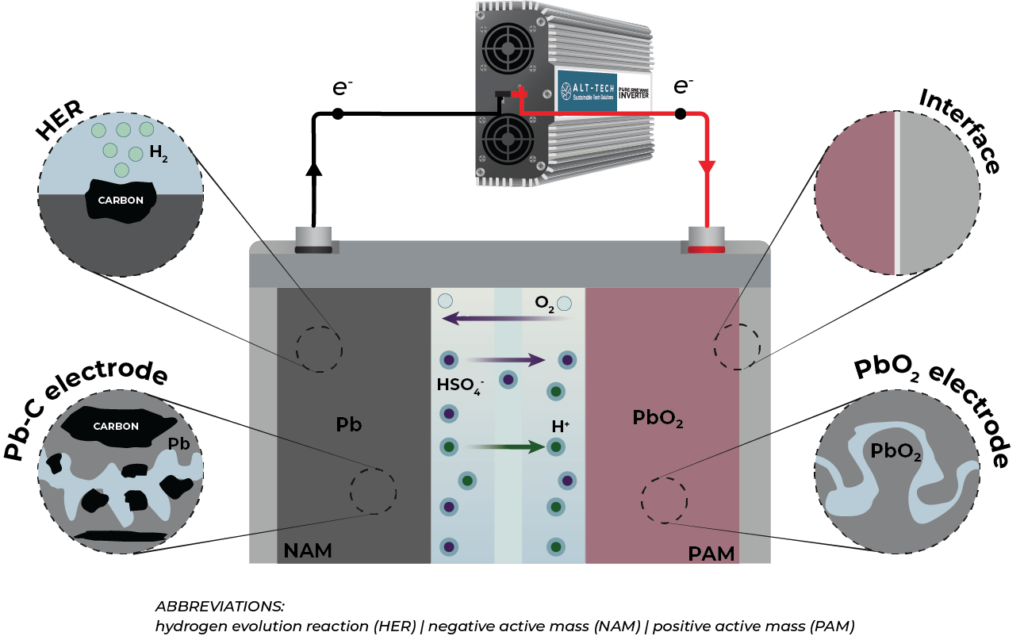
Fig.2. The main obstacles and considerations in lead-carbon batteries. Adapted from [1]
Benefits of Lead-Carbon Batteries
Extended Cycle Life: Lead-carbon batteries offer a significantly longer cycle life compared to traditional lead-acid batteries, incredibly close to nowadays lithium batteries really, making them a cost-effective solution in the long run.
High Charge and Discharge Rates: The incorporation of carbon materials enhances the power density of lead-carbon batteries, allowing for efficient charge and discharge operations, even under high load conditions.
Improved Performance at Low Temperatures: Lead-carbon batteries exhibit a broader operational temperature range compared to standard lead-acid and lithium LFP batteries. This versatility allows lead-carbon batteries to function in a variety of environmental conditions, making them suitable for applications with a wider temperature range.
Enhanced Efficiency and Energy Density: The hybrid design of lead-carbon batteries results in improved efficiency and energy density, contributing to their widespread adoption in renewable energy storage systems.
Electronics-Free Design: A significant advantage of lead-carbon batteries is their simplicity. Unlike modern lithium batteries that often include complex electronic components, lead-carbon batteries maintain a simple design. The lack of electronics decreases the number of things that can fail and increases reliability.
Cost-Effective Energy Storage: Lead-carbon batteries provide a great price per kilowatt-hour (kWh) of usable energy when accounting for both initial cost and expected longevity. This cost-effectiveness makes them a compelling choice for applications where optimizing the balance between performance and budget is crucial.
Environmentally Friendly: With a focus on sustainability and a recycling rate of 97%, lead-carbon batteries stand out as an environmentally friendly option, particularly when compared to the unresolved recycling challenges associated with lithium batteries.
Drawbacks of Lead-Carbon Batteries
Limited Energy Density Compared to Lithium-Ion: While lead-carbon batteries offer enhanced energy density compared to traditional lead-acid batteries, they still lag behind lithium-ion batteries in terms of energy storage capacity by size and weight.
Comparison with Lithium Iron-Phosphate Batteries
Now, let’s compare lead-carbon batteries, specifically the AGM type, with lithium iron-phosphate (LiFePO4) batteries, focusing on key parameters:
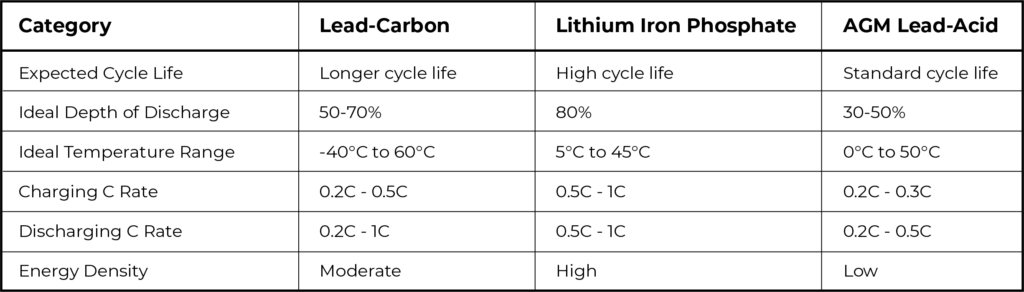
When to Choose Lead-Carbon
In the rapidly evolving landscape of energy storage solutions, lead-carbon batteries (LCBs) have emerged as a formidable option, especially in stationary applications where reliability and longevity are paramount. Their extended cycle life, high charge-discharge rates, and improved performance in ranging temperatures make them a cost-effective and sustainable choice for various energy storage needs.
On the other hand, lithium iron-phosphate (LiFePO4) batteries excel in portable applications where temperature control and space constraints are critical considerations. The high energy density and compact design of LiFePO4 batteries make them ideal for powering portable electronic devices and electric vehicles, providing efficient and reliable performance in situations where size and weight matter.
Ultimately, the choice between lead-carbon and lithium iron-phosphate batteries depends on specific application requirements. Lead-carbon batteries shine in stationary setups, offering robustness and reliability, especially in higher temperature environments. Meanwhile, lithium iron-phosphate batteries carve a niche in portable and space-restricted scenarios, delivering compact and temperature-controlled power solutions. As technology advances, these two technologies will likely continue to complement each other, catering to diverse energy storage needs across different sectors.
Lead-Carbon Batteries
If you’re considering lead-carbon batteries for your system, we have a range of carbonPLUS 2V and 12V batteries available. Each product has a specification sheet if you need to delve into finer details.
carbonPLUS Range
References
[1] Yin, J. Lin, H. Shi, J. et al. Lead‑Carbon Batteries toward Future Energy Storage: From Mechanism and Materials to Applications. Electrochem. Energy Rev. 5, 2 (2022). https://doi.org/10.1007/s41918-022-00134-w
Leave a Reply
You must be logged in to post a comment.